Current funded projects
2024–2024
Towards in situ measurements of iceberg calving at the laboratory scale
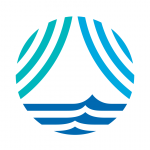
Funding agency: Woods Hole Oceanographic Institution (IR&D #30771; $82k)
Description: Icebergs form via the propagation of through-going fractures, termed rifts, that dissect floating ice shelves along the margins of the Greenland and Antarctic ice sheets. Over the past 50 years, such events have become more frequent, causing the Antarctic ice sheet to retreat by 18%. This trend has severe implications for global sea level rise, since ice shelves provide a buttressing effect that inhibits seaward ice flow. However, ice fracture propagation is poorly understood, making it difficult to account for iceberg calving in ice discharge and sea level rise models. Here, the PI proposes a suite of experiments to parameterize ice crack propagation and fatigue under cyclic stresses that mimic Earth’s tidal forces. Central to this effort will be the development of a novel setup for mapping acoustic emission events (e.g., crack tip propagation) in situ, and in 3-D, within the ice samples.
2023–2026
Microstructural evolution during superplastic ice creep
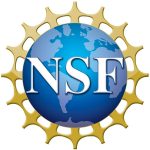
Funding agency: National Science Foundation (OPP-2317263; $494k)
Description: Ice flow is predominately modeled using a constitutive equation termed “Glen’s law”, which was constrained under relatively high-stress conditions and describes ice flow rate as a function of temperature and stress. Glen-type flow is usually attributed to the motion of crystal defects within ice grains. However, experiments performed on fine-grained samples suggest that under low stress "superplastic" conditions, grain boundary sliding (GBS) becomes the rate-controlling process. The grain boundary sliding hypothesis is contentious because GBS is not thought to produce a crystal orientation fabric (COF)—that is, an alignment of ice crystals during flow—whereas polar ices widely exhibit strong COFs. Crucially, however, very few COF measurements have been conducted on ice samples deformed under superplastic flow conditions in the laboratory. The primary goal of this project is to measure the evolution of ice COF across the transition from superplastic to Glen-type creep. Results will be used to interrogate the role of superplastic GBS creep within polar ice masses, and thereby provide constraints on polar ice discharge models.
2022–2025
Strength of the oceanic lower crust: new experimental and microstructural constraints
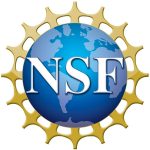
Funding agency: National Science Foundation (OCE-2103031; $641k)
Collaborators: Veronique Le Roux (WHOI), Rellie Goddard (University of British Columbia)
Description: Along slow- and ultraslow-spreading mid-ocean ridges, new ocean crust is formed through the motion of large "detachment faults", which act as vast geologic conveyor belts, bringing rocks from the deep crust and upper mantle to Earth's seafloor. However, there is long-standing debate over whether such faults nucleate in the shallow subsurface, due to water-rock reactions, or in the lower crust, due to viscous shear weakening. In large part, this debate reflects uncertainty over the strength of the viscous lower crust. To resolve this debate, we are conducting a series of high-temperature, high-pressure experiments on lower crustal samples. From the experiments, we will quantify the stress-dependence of various rock properties—namely, grain size and subgrain size—and apply those findings to lower crustal rocks obtained from the Southwest Indian Ridge via drilling.
2020–2024
Funding agency: National Science Foundation (EAR-2023128; $542k)
Collaborators: David Goldsby (University of Pennsylvania), Lars Hansen (University of Minnesota)
Description: Most minerals undergo phase transformations—changes in their crystal structure—as they are subjected to extreme changes in pressure and temperature. Phase transformations have been invoked as an important source of mechanical weakening during mountain building, subduction, and mantle convection. However, such weakening, which is short-lived by definition, has long proven difficult to capture in the laboratory. This project involves high-pressures, high-temperature experiments in a D-DIA apparatus at the Advanced Photon Source synchrotron. By leveraging sychrotron X-ray radiation, we can directly observe and quantify stress, strain, and crystal structure in situ, and thereby resolve transformation-induced weakening.