Penguin Life Observatories
Penguins in the Southern Ocean
Understanding, mitigating and reversing human-induced loss of biodiversity is one of the most important and urgent scientific, economic and ethical challenges we face today. Our long-term research goal is to advance our understanding of ocean ecosystems within a movement ecology framework. We are especially interested in understanding the impacts of global change on the Southern Ocean's ecosystem, where remote sensing methods enable us to study sparse top predator populations. As top predators, we study three different penguin species, emperor penguins, king penguins and Adelie penguins.
Combining methods from statistical physics, computer vision and movement ecology, we strive to advance methods and technologies across the spectrum from detecting presence and animal counting to remote sensing of behavior and life history. For example, we study emergent features of collective behavior to estimate energy budgets in penguin colonies. Remote sensing methods allow us to understand remote ecosystems that are under significant pressure from global change, but at the same time difficult to access, so they remain understudied.
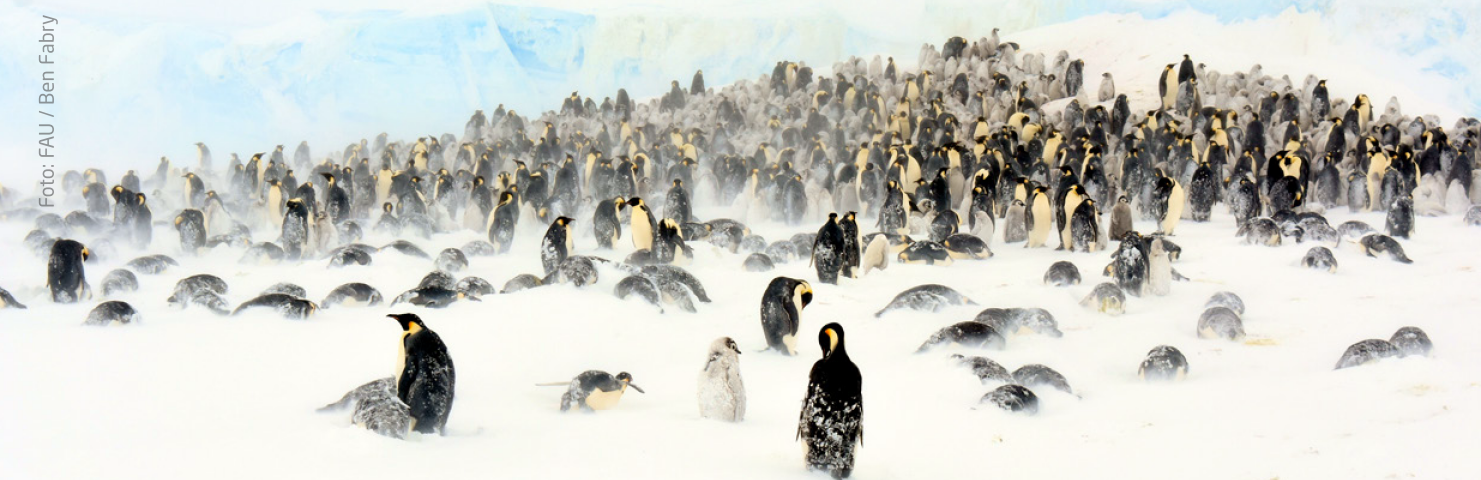
Current Projects
MARE (Monitor the health of the Antarctic maRine ecosystems using the Emperor penguin as a sentinel) is a long-term monitoring program (30 years duration) that assesses the vulnerability of Antarctic ecosystems using the Emperor penguin as sentinel species. This second-worldwide Life Observatory of emperor penguins (started in 2017) aims to predict the species' adaptive potential to climate change and associated fluctuations in prey abundance and distribution. As umbrella species, seabirds can play an important role in determining the size for Marine Protected Areas (MPAs), and help to map marine biological hotspots. Knowledge of the distribution at sea and foraging strategies of emperor penguins is extremely scarce. Life-long monitoring of the birds at their colony is performed using Passive
Integrated Transponders (PIT) and Radio-Frequency Identification (RFID) systems. At sea monitoring is conducted by biologging of birds from Atka Bay colony (multi-sensor and satellite recorders) at different stages of their life cycle, and over regular intervals (e.g. 5 years). The RFID and biologger-tagged birds need to be scanned and/or recaptured.
Currently, we are optimizing and automate the data retrieval process by using an autonomous and remote controlled unmanned ground vehicle (UGV) as an automatic data-downloading terminal. Mounted with wireless data-receivers, the ECHO UGV will automatically retrieve oceanographic data from equipped birds. This has the advantage that we do not miss the chance of collecting data during the short intervals when the birds are present at the colony to feed their chick (which has been the case up-to-now), and to limit the animals' disturbance by reducing the number of birds' recaptures and the human presence at the colony site that was previously required for the time-consuming and difficult search for the equipped birds out of a crowd of up to 20 000 birds.
SPOT (Single Penguin Observation and Tracking) was initally designed and deployed (2013) to study collective behavior in emperor penguin colonies, and became an integral part of the MARE program since its inception. It is our most advanced remote controlled Emperor Penguin observatory. It is deployed next to the Atka Bay emperor penguin colony, close to the German Antarctic research base Neumayer Station III. SPOT serves multiple purposes. Intended for studying emergent phenomena during huddling of Emperor Penguins during Antarctic winter. SPOT is equipped with 16 cameras with different resolutions and sensors, allowing to record low and high resolution images of the location penguin colony, as well as of thousandths of individual penguins at the same time. Tracking of individual penguins as well as the whole colony allows us to unravel the mechanisms how emperor penguins coordinate themselves, react and adapt to different environmental conditions.
Collective Behavior in Emperor Penguin Colonies
Huddles are dense formations of penguins, with often more than hundreds of individuals, reaching densities of up to 10 birds/m2 (2). Inside a huddle, ambient temperatures are above 0 °C (3) and can even reach above 20 °C (2). Penguins on the windward edge of a huddle feel the cold more than those in the center and down-wind, and therefore need to move somehow to the huddle center. Huddling poses an interesting physical problem.
Questions we aim to answer with this project include: How do huddles move as a whole, and how do penguins move within a huddle (relative to their neighbors), if at all? How is the time spent inside the huddle distributed among individuals? How do individuals join, break loose and rejoin the group, and what effect on the energy budget does this have? Does huddle formation and fusion depend on colony size, and how does it depend on the weather conditions? Other "byproducts" of the data, e.g. body mass, will elucidate the effects of the nutritional state on huddling behavior.
Remote sensing of Emperor Penguin foraging success
Using time-lapse imagery, we are developing methods to remotely sense foraging success of the whole colony after the penguins return from foraging to breed. By correlating the penguin's "wind chill" temperature with video observations of when the penguins begin huddling, we are able to come up with a "transition temperature" - the temperature at which colonies shift from a scattered, liquid-like state to a huddled, solid-like state. If the transition occurs at warmer temperatures, it means the penguins are feeling cold earlier and begin huddling to stay warm and conserve energy. And that indicates that the penguins had less body fat upon their return from foraging and were probably undernourished because they did not find enough food to eat within a reasonable distance from their breeding colony. If the transition temperature is lower later in the season, it suggests that the foraging season was a success and the animals returned well-fed and with higher amounts of body fat. This proof of concept is currently expanded to larger datasets spanning almost a decade.
King Penguin Colony Organization
King Penguins live in large colonies with up to several ten of thousand of individuals. The colonies can be in different states, from a uniform mixture of adult and chick penguins to a highly phase separated state where chicks move collectively. Using non-invasive time-lapse photography we study the underlying rules of colony organization. We derive near physics models to explain how such complex organsiztional feature can emerge from simple animal - animal interaction. Current observatories (micr0bs) are based on Crozet and Kerguelen Island (with a lot of help from IPEV and CNRS) and record continuous time-lapse images at different time intervals (from 30fps to 1 fpm) allowing us to study the organizational processes on different time scales.
Gutt J, Arndt S, Bornemann H, Eisen O, Flores H, Griffiths H, Hattermann T, Hain S, Hoppema M, Isla E, Janout M, Mark FC, Moreau S, Trimborn S, van Opzeeland I, Pörtner HO, Zitterbart DP, Piepenburg D „ A roadmap to observe, understand, and project ecosystem response to environmental change in the East Antarctic Southern Ocean”, https://bg.copernicus.org/preprints/bg-2022-110/ (submitted to BioGeoSciences, May 2022)
*Houstin A, Zitterbart DP, *Winterl A, *Richter S, Planas-Bielsa V, Chevallier D, Ancel A, Fournier J, Fabry B, Le Bohec C, "Biologging of emperor penguins - attachment techniques and associated deployment performance", (preprint), https://www.biorxiv.org/content/10.1101/2021.06.08.446548v1
*Houstin A, Zitterbart DP, Heerah K, Eisen O, Planas-Bielsa V, Fabry B and Le Bohec C, "Current conservation measures do not provide effective protection for emperor penguins." (preprint), https://www.biorxiv.org/content/10.1101/2021.04.06.438390v1.full
Labrousse S, Iles D, LaRue M, Viollat L, Fretwell P, Trathan P, Zitterbart DP and Jenouvrier S. “Quantifying the causes and consequences of variation in satellite-derived population indices: a case study of emperor penguins”, Remote Sensing in Ecology and Conservation (2021), http://doi.org/10.1002/rse2.233
*Winterl A, *Richter S, Houstin A, Nesterova AP, Bonadonna F, Schneider W, Fabry B, Le Bohec C and Zitterbart DP. micrObs, a low-cost mobile camera observatory for observation of dynamic processes in animal groups. HardwareX, 20 August 2020, e00134, https://doi.org/10.1016/j.ohx.2020.e00134
Trathan PN, Wienecke B, Barbraud C, Jenouvrier S, Kooyman G, Bracegirdle TJ, Phillips T, Le Bohec C, Ainley D, Ancel A, Zitterbart DP, Chown S, LaRue M, Cristofari R, Younger J, Clucas G, Bost CA, Brown J, Gillett HJ, Fretwell PT. The emperor penguin - vulnerable to projected rates of warming and sea ice loss. Biological Conservation, 2019; 108216, http://doi.org/10.1016/j.biocon.2019.108216
*Gerum R, *Richter S, *Winterl A, Mark C, Fabry B, Zitterbart DP. CameraTransform: A Python package for perspective corrections and image mapping. SoftwareX, Volume 10, 2019, 100333, ISSN 2352-7110, https://doi.org/10.1016/j.softx.2019.100333
*Richter S, *Gerum R, *Winterl A, Houstin A, *Seifert M, *Peschel J, Fabry B, Le Bohec C and Zitterbart DP. Phase transitions in huddling emperor penguins. J. Phys. D: Appl. Phys. 51 214002 (2018) https://doi.org/10.1088/1361-6463/aabb8e
*Gerum R, *Richter S, Fabry B, Nesterova A, Le Bohec C, Bonadonna F, and Zitterbart DP. Structural organisation and dynamics in king penguin colonies. J. Phys. D: Appl. Phys. 51 164004 (2018), https://doi.org:10.1088/1361-6463/aab46b
*Richter S, Gerum R, Schneider W, Fabry B, Le Bohec C, and Zitterbart DP. Remote controlled observatories for behavioral and ecological research on seabird colonies. Methods in Ecology and Evolution, (2018), https://doi.org/10.1111/2041-210X.12971
*Gerum R, Richter S, Fabry B, and Zitterbart DP ClickPoints: an expandable toolbox for scientific image annotation and analysis. Methods in Ecolology and Evolution, MEE (2016), https://doi.org/10.1111/2041-210X.12702
Cristofari R, Bertorelle G, Ancel A, Benazzo A, Le Maho Y, Ponganis PJ, Stenseth NC, Trathan PN, Whittington JD, Zanetti E, Zitterbart DP, Le Bohec C & Trucchi E. Full circumpolar migration ensures evolutionary unity in the Emperor penguin. Nature Communications, 7:11842, https://doi.org/10.1038/ncomms11842 (2016).
Zitterbart DP, Richter S, Spiekermann G, Behrens LK, Regnery J, Fontes RP, Hänssler T, König-Langlo G, Weller R, Fabry B. Are environmental factors responsible for changed breeding behaviour in emperor penguins? Antarctic Science, 26, pp 563-564, 2014., https://doi.org/10.1017/S0954102014000285
*Gerum R, Fabry B, Metzner C, Beaulieu M, Ancel A, Zitterbart DP. Origin of traveling waves in an emperor penguin huddle. New Journal of Physics 15 (2013) 125022 (17pp), https://doi.org/10.1088/1367-2630/15/12/125022
Zitterbart DP., Wienecke B, Butler JP, Fabry B. Coordinated movements prevent jamming in an Emperor penguin huddle. PLoS ONE 6(6): e20260. https://doi.org/10.1371/journal.pone.0020260 (2011)