Buoyant Boundary Currents
Buoyant coastal currents transport freshwater, heat, nutrients, sediments, pollutants, and biological organisms along many continental shelves and have important impacts on ecosystems, fisheries, and coastal circulation. On the global scale, buoyant coastal currents are primarily responsible for the redistribution of freshwater. Considerable work is needed to improve our understanding of the pathways and mechanisms by which buoyant coastal currents adjust to coastline and topographic changes and the dynamics by which they move away from the coast. I have focused on several aspects of boundary current dynamics, and recently investigated the effects of coastline changes and the interaction of multiple currents of different densities.
When a slope-controlled buoyant coastal current encounters a canyon, the current separation at the upstream wall of the canyon depends on the ratio of the current width to the radius of curvature of the bathymetry (Sutherland & Cenedese 2009). The current moved across the mouth of the canyon for values of the ratio larger than unity. The significant result of this study, carried out in collaboration with Dave Sutherland (MIT-WHOI Graduate Student), is that, although bottom friction is important in setting the position of the buoyant front, the separation process is driven by the inertia of the flow. These results support the observational driven hypothesis that the path of the East Greenland Current is strongly influenced by the shelf bathymetry.
Similarly, when a buoyant coastal current crosses a gap in the coastline, the fraction of buoyant water that enters the gap has been found, in the laboratory, to depend on the ratio of the channel width to the Rossby radius of deformation. This is true both for surface-trapped and slope-controlled currents. However, the details are different for the two kinds of currents. A slope-controlled current can cross the gap less easily than a surface-trapped current, with a consequent increase of the buoyant flux into the gap. For gaps with a sharp corner, an eddy was observed to form and it allowed the current to “leap-frog” the gap with a consequent reduction of buoyant fluid entering the gap. This results of this project were found in collaboration with Michelangelo Mariani, Michela De Dominicis, and Igino Angelini (Undergraduate Guest Students) and will be reported in two manuscripts in preparation.
Boundary current dynamics become more complicated when multiple buoyant sources having different densities are present. One relevant example of this scenario is the Gulf of Maine, where multiple rivers combine to form the western Maine coastal current. Even the simpler problem of how two buoyant currents align vertically and horizontally after they adjust to be in geostrophic balance, is still poorly understood. A combination of laboratory experiments and analytical calculations in collaboration with Jim Lerczak (Oregon State U.), Riccardo D’Andrea and Valentina Dore (Undergraduate Guest Students), has recently revealed that the horizontal and vertical alignment of the two currents depends on the ratio of the Rossby radii of deformation of the two water masses. Ongoing laboratory experiments in collaboration with Giuseppe Bartone (Undergraduate Guest Student) confirm the analytical model results and reveal interesting “coupled” frontal instabilities. Together with Domenico Mussardo and Yackar Mauzole (Undergraduate Guest Students), we compared these instabilities with single frontal instabilities previously observed by Griffiths et al. (1982) and Griffiths & Linden (1981). These results are documented in Cenedese & Lerczak (2007) and in two manuscripts in preparation.
In collaboration with Mike Spall (WHOI) I have been investigating the downwelling concentrated in strong currents subject to buoyancy loss near lateral boundaries. Theoretical understanding of what controls the downwelling near boundaries (its magnitude, length scales) is weak and relies on parameterizations of poorly known turbulent mixing processes. There is a lack of clear understanding on whether the downwelling occurs over the spatial scale of the boundary current or within a narrower boundary layer. I conducted laboratory experiments to resolve the turbulent mixing due to convective plumes and identify where downwelling takes place in order to determine whether the details of these small-scale turbulent processes need to be resolved explicitly in order to represent their influence on the larger-scale circulation.
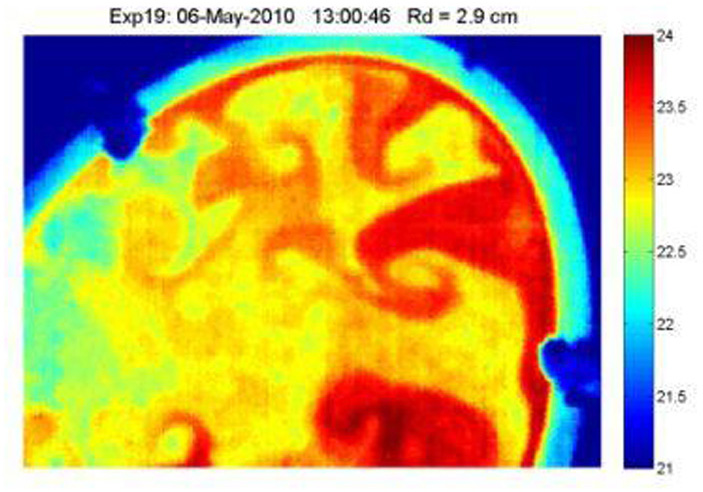
Surface temperature (°C) of a current subject to buoyancy loss near lateral boundaries.
References
Cenedese C., and Lerczak J.A., 2007. Understanding the dynamics of the interaction between two river plumes. Fifth International Symposium on Environmental Hydraulics, ASU, AZ.
Griffiths, R.W., Killworth, P.D., and Stern, M.E., 1982. Ageostrophic instability of ocean currents. J. Fluid Mech., 117, 343-377.
Griffiths, R.W., and Linden, P.F., 1981. The stability of vortices in a rotating, stratified fluid. J. Fluid Mech., 105, 283-316
Sutherland D.A., and Cenedese C., 2009. Laboratory experiments on the interaction of a buoyant coastal current with a canyon: application to the East Greenland Current. J. Phys. Oceanogr., 39, 1258-1271.