Background
Indian Ocean meridional overturn
The Indian Ocean converts deep and bottom water to intermediate and thermocline waters that emerge and join either the upper part of the Atlantic’s overturn or the low oxygen upper portion of the Circumpolar Deep Water in the Antarctic, where they eventually upwell to the thermocline (Figs. 1.1). The Pacific Ocean’s role is parallel to the Indian Ocean’s; they are further coupled to each other through the upper ocean’s Indonesian Throughflow (ITF). The ITF itself enters the Indian Ocean with a set of properties set by the Pacific and conditions within the passages, then is modified as it transits the Indian Ocean; the balancing transport that exits the Indian Ocean in the upper layers of the Agulhas has different properties from the inflow, so there is an ITF impact on all property divergences/fluxes within the Indian Ocean. Furthermore, Indian Ocean overturning cells are important for redistribution of heat and other properties, including importantly a very shallow cross-equatorial cell (Schott et al., 2002); it involves the important Arabian Sea surface upwelling center, influencing air-sea fluxes such as carbon and oxygen, as well as being influenced by air-sea fluxes of heat and freshwater. The standard subtropical gyre cell is also important for heat and freshwater movement, and is the main vehicle for importing freshwater to the Indian Ocean from the south (Talley, 2008).
There is a wide array of deep-to-shallow Indian Ocean overturn estimates, ranging anywhere from 2 to 30 Sv (1 Sv = 1x106 m3s-1), with most likely size from 10-17 Sv (Fig. 1.2). It is expected that the carbon transport and, particularly, anthropogenic carbon transport will be sensitive to the structure and magnitude of the overturn. The deep to shallow basin-scale upwelling points to the importance of good estimates of vertical transport and diffusivity in understanding property budgets in this basin. The large range of overturn estimates begs to be narrowed with application of the best available data sets and tools.
Overturn estimates have focused on the subtropical 32°S section because it is the southernmost land-to-land section in the Indian Ocean, thus constraining budgets for all of the Indian Ocean. However, the upwelling and mixing processes are obviously not uniform throughout the Indian Ocean, just as the surface forcing is not. The meridional overturn portion of our study includes close attention to partitioning the Indian Ocean in latitudinal bands, to study the relative importance of the major north-south regions: north Indian Ocean, tropics including the equator, South Equatorial Current including the Indonesian Throughflow component, and the subtropical gyre.
Deep upwelling occurs throughout the Indian Ocean, although there is strong new evidence of dependence on mid-ocean ridges (Drijfhout and Naveira Garabato, 2008; section 1.2 below). Shallow upwelling is strong in the tropics, and especially in the Arabian Sea. Resolution of the tropical shallow overturning cell (Schott et al., 2002) is important. Because time dependent forcing, circulation, and eddies are intrinsic to this cell, the POP and ECCO-GODAE analyses of the cell and its transports are essential.
Indian Ocean diapycnal diffusivity is inhomogeneous (section 1.2 below; Kunze et al., 2006; Mackinnon et al., 2008). But diapycnal upwelling also depends on the vertical property structure, so the mixing inhomogenity may differ from that of diffusivity which is highest over ridges with significant tidal fluxes and around Kerguelen (Kunze et al., 2006). However, it appears that the tropical band has greater diapycnal mixing (Talley et al., 2003). This is an important issue, with the first step to be resolved when looking at meridional overturn, but necessarily followed by a regional analysis that defines ridge and boundary regions.
Heat and freshwater transports
The Indian Ocean gains heat north of about 20 °S and the ITF transports warm upper ocean water into the Indian Ocean at about 12 °S. This heat must be exported southwards. At 32°S, the net southward heat transport is order 1 PW, with a wide range of estimates, as with volume transport (e.g. Ferron and Marotzke, 2003). Talley (2008), with a net of 0.8 PW, found that less than half of the total (~0.3 PW) is carried by the upper Indian Oceans’s subtropical gyre; half (0.4 PW) is associated with the overturning from deep to intermediate/thermocline waters, and the remainder with the change in temperature of the ITF as it passes through the Indian Ocean (0.1 PW) (e.g. Talley, 2008). The deep upwelling is therefore significant for the global heat budget. For freshwater (FW), the deep upwelling is less significant, while the upper ocean (gyre and ITF) carries much of the northward FW transport required to balance net evaporation in the Indian Ocean (Talley, 2008). While almost all budgets have been constructed with most attention given to the 32°S section (Fig. 1.1), there are significant meridional variations in freshwater input in the Indian Ocean. What seems most curious about the wide ranges of estimates is that most are based on the same data sets, so sensitivity to choices such as the magnitude of the ITF is important.
In the northern Indian Ocean, net heat gain in the warm pool must be balanced by southward export of heat across the equator and out of the tropics (e.g. Wacongne and Pacanowski, 1996; Loschnigg and
Webster, 2000). Upwelling of deep to thermocline waters remains vigorous even north of the equator, and maybe be responsible for a significant fraction of the heat export. The shallow equatorial ‘roll’ circulation is strongly time dependent, with monsoonal reversals in currents and shallow upwelling; the annual mean is dominated by the SW monsoon conditions (Schott and McCreary, 2002) and exports heat southwards. This same pair of mechanisms (shallow roll and deep upwelling) impacts all of the other properties (carbon, oxygen, freshwater). In the tropics, resolution of the very large time dependence is essential to quantifying the relative importance of the deep and shallow cells for exporting heat, and hence to the sensitivity of the heat budget to interannual and decadal variability in surface forcing (e.g. Indian Ocean Dipole, ENSO, climate change). Even the deep cell is likely to depend on surface forcing if the mixing processes are associated with deep reversing currents and planetary waves that continually adjust the circulation. We propose to work intensely with the POP model output on this aspect of the Indian Ocean overturn, with comparisons with the snapshot hydrographic analysis to assess validity of the deeper parts of the modeled circulation.
Carbon transports
The Indian Ocean represents a net source of CO2 to the atmosphere (Bates et al. 2006), while simultaneously storing approximately 20% of the total global inventory of anthropogenic carbon (Sabine et al., 2004) (Figure 1.1b). A number of investigations (Holfort et al., 1998; Roson et al., 2002; Alvarez et al., 2003, Macdonald et al., 2003) have used observations from long transects to understand how the overturning circulation in the Atlantic affects the transport, divergence and air-sea flux of carbon within that basin. Although similar work has considered the Indian Ocean as part of a global product (Mikaloff-Fletcher et al., 2006; Gruber et al., 2009), none has as yet focused on the interaction between the horizontal and vertical circulation and the natural and anthropogenic carbon budgets in this unique basin.
Much of what is known about the Indian Ocean carbon cycle has been obtained through numerical models, indirectly through atmospheric measurements, and more directly through inventory budgets (Sabine et al. 1999, 2004; Sabine and Feely, 2001; Sarma et al. 2003; Bates et al. 2006). Others (Gloor et al., 2003, Hall and Primeau, 2004; Mikaloff-Fletcher et al., 2006; Gruber et al., 2009) have combined GCM dynamics with the ocean carbon parameter distributions to focus on the latitudinal and air-sea exchanges of observed total inorganic carbon (TIC), and estimated anthropogenic carbon (Cant) (e.g. Gruber et al., 1996) and natural carbon. Thus far, all use of the observed fields for investigating the effects on the flow field of the carbon budget has been limited to the zonal lines in this basin known for its zonal asymmetries. None have as yet used observed dynamics in both hemispheres of the Indian Basin together with the measured TIC and estimated Cant to investigate the relationship between carbon source/sink regions and the geographical distribution of overturn and mixing.
Science questions related to Indian Ocean overturn to be considered by this study
1. Why is the range of overturning transport estimates for section data at 32°S so large? Some possibilities that will be tested are: (a) Sensitivity to the ITF transport. (b) Sensitivity to details of the lateral circulation within isopycnal layers, especially in the intermediate and deep waters (AAIW layer with northward flow from Southern Ocean and southward flow of upwelled deep water; NADW/IDW/CDW layer with northward flow of both NADW and CDW, southward flow of IDW).
2. Why are GCM and other model-based estimates of Indian Ocean overturning low compared with most estimates based on hydrographic data? Some possibilities to test are: (a) Difficulties with flow through narrow gaps between deep basins, with concomitant high mixing in the gaps. (b) Inaccurate parameterization of deep diffusivities with overly-simplified profiles that are too weak or too uniform.
3. For meridional overturning, which broadly defined latitude bands are more effective diapycnal mixing locations? Where is the product of regionally dependent diffusivity and regionally dependent vertical property curvature highest? Can deep-reaching reversing currents and eddies in the presence of rough bottom topography, such as in the tropics and north Indian Ocean, create mixing, much like tidal forcing?
4. Can eddy-driven diffusion (Wolfe et al., 2008) enhance the diffusivity parameterized from ADCP observations (Kunze et al., 2006)? Can lateral stirring by vigorous eddies and reversing currents, especially near fronts where vertical gradients can create hot spots of diapycnal processes?
5. The POP model shows strong localized upwelling at the equator at all depths. What are the dynamics of this upwelling? What is special about the equator that is not captured in observations of diffusivity (Kunze et al., 2006) itself?
6. What balance of mechanisms transport heat southwards out of the tropical Indian Ocean? To consider: (a) Deep overturn with downward diffusion of heat in the northern Indian Ocean, (b) Shallow cross-equatorial cell with northward colder flow in the western boundary current and warmer wind-driven southwards flow across the equator, more in mid-ocean (Wacongne and Pacanowski, 1996; Schott et al., 2002), (c) “eddy” flow associate with the large monsoonal variability, especially in the western Indian Ocean, with reversing currents, reversing eddies, and vigorous Rossby/Kelvin/Yanai wave activity.
7. What is the balance of mechanisms for the same questions for carbon transport, given that there is net outgassing in the tropical and northern Indian Ocean? What portions of the circulation transport carbon most vigorously northwards? And the same questions for freshwater transport, given that the Indian Ocean north of 32°S is net evaporative.
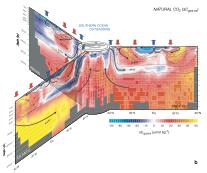
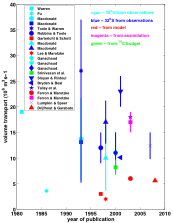
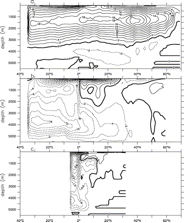